2.4 Obesity
Obese and diabetic people are subject to more infections than healthy people, but interestingly it’s not the body mass index that drives this, but rather the accompanying hyperglycemia.69
2.4.1 Other conditions
Some people claim you can treat migraine headaches, sinusitus, and other conditions by inserting Lactobacillus-containing Kimchi up the nostril. I’ve not tried it and can’t vouch for it, but let me know if it works for you.
2.4.2 Hygiene**
Now for some speculation. I can’t prove any of this, but these are some questions possibly worth asking:
What does (underarm) deoderant do? Although rich people soaked themselves with various colognes for thousands of years, the widespread use of deoderant is less than 100 years old.
Why do you have sweat glands there in the first place? Sweat is supposed to help cool your body, but not much cooling will happen from a surface that’s not exposed to much air. Instead, those glands feed bacteria,
Similar to the way some people do fecal transplants to modify their gut bacteria, someday it may be possible to do arm pit bacteria transplants.
How often should you brush your teeth?
Washing your hands in a public restroom
In Westernized countries for the past hundred years, it’s been taken for granted that everyone should wash their hands every time they use the toilet, especially in a public place. Washing with soap and water, of course, is an effective way to remove harmful microbes, but I wonder what happens to your hands after washing, when you turn off the faucet or help yourself to the paper towel machine. Even in a restroom with those fancy automatic on/off devices, you still probably touch the doorknob on your way out.
We know that fecal matter often harbors pathogenic microbes, and of course the act of doing your business makes it likely that your hands will come closer to unpleasant waste products, but I wonder: most of the microbes you’re touching are already on your body. I suspect that microbes optimised for some parts of your body won’t do well on the hands anyway. But meanwhile, that faucet is being handled all day by crowds of strangers, each hosting microbes that probably enjoy the moist, pleasant surfaces of the bathroom sink.
Isn’t it better to enter and leave the public restroom without touching anything other than yourself?
If you do wash your hands in the restroom, what’s the best way to dry your hands afterwards? The short answer: paper towel: the friction from rubbing your hands helps loosen and dislodge microbes, while an air dryer can just spread more germs into the air. If you’re concerned about the environment from all that paper towel waste, a careful analysis of the tradeoffs shows that both methods are about equivalent once you take into account manufacturing, installation, electricity, and final disposal costs.
How to wash food in the kitchen
By far the most common source of pathogens in food happens during meal preparation. This makes it tempting to wash everything over and over throughout the cooking process, but be careful: you might just be splashing those microbes onto other surfaces throughout the kitchen.
If you wash a whole chicken in your sink, for example, any germs on the exterior will unavoidably find themselves in tiny water molecules that may land anywhere in the kitchen. You’ll scrub the counters afterwards, of course, but beware that it’s hard to disinfect everywhere: the curtains? the ceiling?
Like most battles with microbes, there’s a tradeoff between killing large numbers in a visible way (“carpet-bombing”) and preventing them from gathering in volumes in the first place.
Generally it’s best to leave food untouched if you’ll be cooking it. Fruits and vegetables meant for eating raw should be cleaned, but just rinsing with water isn’t going to dislodge the serious pathogens. Rinsing in vinegar or other acidic chemicals can kill most of the germs, at the expense of some flavor.
Ultimately the only sure way to beat pathogens in the kitchen is through high heat, so if you have reason to suspect that your food might be contaminated, you should cook it.
The dangers of kitchen sponges
Microbes and cooking
Few microbes that inhabit human bodies are able to survive long at temperatures over 165 degrees (F).
2.4.3 Diversity and health
Most microbiome discussions begin with the assumption that diversity is good. Virtually any popular book or article about how to improve your health will suggest ways to increase diversity, usually by eating specific foods. For what it’s worth, a study of more than 10,000 gut microbiomes found only one sure-fire association with higher gut diversity: people who self-report eating more plants have higher diversity than those who eat fewer types of plants, and this is true no matter their diet type (omnivore, vegan).70
The intuition is easy to understand: if your body harbors a wide variety of microbes, you’ll have a deeper catalog of useful ones that can be applied to new situations. The world around us is constantly changing, and you never know what new threats or opportunities you may encounter. You can respond better if you have an abundant variety of organisms that can meet any challenge.
In practice, diversity is difficult to pin down quantitatively. We know what we mean in principle: having a variety of different microbes seems good, but clearly there are limits. You wouldn’t want “variety” to include serious pathogens, for example. We know intuitively that a deciduous forest at sea level, with dozens of differerent tree species, is more diverse than one at a high altitude tree line. But is the one at low altitudes “better”? It depends on where you live!
A similar problem has long confronted ecologists, who have developed several diversity measures that have been adopted to the micro world:
Alpha diversity: the variance within a particular sample. Usually measured as a single number from 0 (no diversity) to infinity, or sometimes as a percentile, this is what most of us mean when we look at our microbiome results and ask about diversity.
Beta diversity: how samples vary against each other. Many scientific studies are interested in the differences between sites on the body, or microbiomes across geographic locations. Beta diversity is typically the thinking behind “clustering” algorithms that try to show differences or similarities among samples.
All diversity metrics take into account two aspects of a community: the number of different organisms in a sample, and the range of abundances for each one. To understand how this works, think of two forests, each with an equal number of trees. (Table 2.1)
Forest | Number of Trees | Number of Species | Diversity |
---|---|---|---|
A | 1000 | 1 | Low |
B | 1000 | 1000 | High |
C | 10 | 10 | ? |
Clearly, Forest B with its abundance of species and trees is the most diverse. But what about Forest A compared to Forest C?
On the one hand, Forest C seems to have a greater variety of trees: 10 times more than Forest A. But it also has many fewer of them. In other words, there are two aspects of diversity that matter: the absolute number of organisms in an ecosystems, and the variety or richness of those that are there.
Whether A is “better” or “worse” than C depends on subjective, non-quantifiable factors that are not included in any diversity metric. A managed forest, such as one on a Christmas tree farm, might be perfectly healthy for one purpose (growing Christmas trees for sale), while an adjacent clear-cut forest with ten lonely and scraggly trees could be far less healthy, even if it has more of a variety of trees.
In this example, we use the distinction richess to refer to Forests B, or C, with their greater variety of species, and the overall term diversity tries to be a measure of both richness and abundance.
We can apply the same principle to our taxonomy tables: A microbiome sample with 100 unique taxa is more diverse than one with only 10 unique taxa. But if we just use raw, absolute numbers, it can be hard to compare across different microbiome tests. For example, what if I have two samples, each with 100 unique taxa, but in one sample there are tiny amounts of all but one of the taxa, while the other sample has equal amounts of everything? Which is more diverse?
One way to quantify this is with a metric borrowed from probability theory. What if, instead of looking at all the taxa and their respective amounts, we simply take at random any two taxa from the sample: what is the probability that the two will be the same?
If I have a sample with 100 unique taxa, each of identical abundance, then the odds are pretty low that I would select at random two of the same taxa; conversely, if a majority of the sample consists of the same taxa, with many other taxa of smaller abundance, then the odds are pretty good that the two I select would be the same.
In fact this is generally the case in healthy western guts, which are usually composed of only two large phyla: Firmicutes and Bacteroidetes. In my case, as you’ll see, these two phyla make up over 90% of everything I see in my samples; the third most abundant taxa rarely breaks 10%. The odds that you would randomly pick these two is pretty high. That’s the intuition behind the Simpson metric, developed in 1949 by the British scientist E.H. Simpson.
But note that with Simpson, high numbers mean low diversity; after all, in a homogeneous sample with no diversity, the odds that you’ll pick the same taxa will be 100%. To keep this consistent with the idea that higher numbers mean more diversity, most scientific studies of the microbiome use Inverse Simpson, which is simply 1 divided by the Simpson number. Note that for very low Simpson numbers, the Inverse Simpson value can be quite high, even approaching infinity when dealing with a microbiome with many unique and extremely low abundance taxa.
The taxonomy of microbes matters too. Each successively lower taxonomical rank always has at least as many taxa as the higher levels, so you can’t simply count the total number of taxa at a rank. A single genus like Bifidobacterium, for example, can have dozens of species associated with it. For this reason, microbiologists usually measure diversity at the Family level: it’s a good compromise between overall coverage and specificity of taxa.
In the real world, the type and variety of microbes in the body are constantly changing, so it’s important not to get too hung up on a single number for a single sample. You’ll see this later when we look at how diversity changes in my own experiments
The key is to take multiple samples and not rely on a single day’s measure. If you take many samples over time, you’ll find that the moving average is much more stable, and a better overall indicator of diversity.
There are other measures of diversity as well. The Shannon Index borrows from Information Theory to ask how much unique information is contained in a given sample. A radio signal that broadcasts random static, for example, would have a lower Shannon number than one for a music concert. Similarly, a microbiome a boring makeup – all the same species, for example – would have a lower Shannon number than one containing a rich abundance of many different types of microbes and abundances. In practice, Shannon and Inverse Simpson tend to track one another reasonably well, a clue that they are getting at a similar idea. (Figure 2.5)
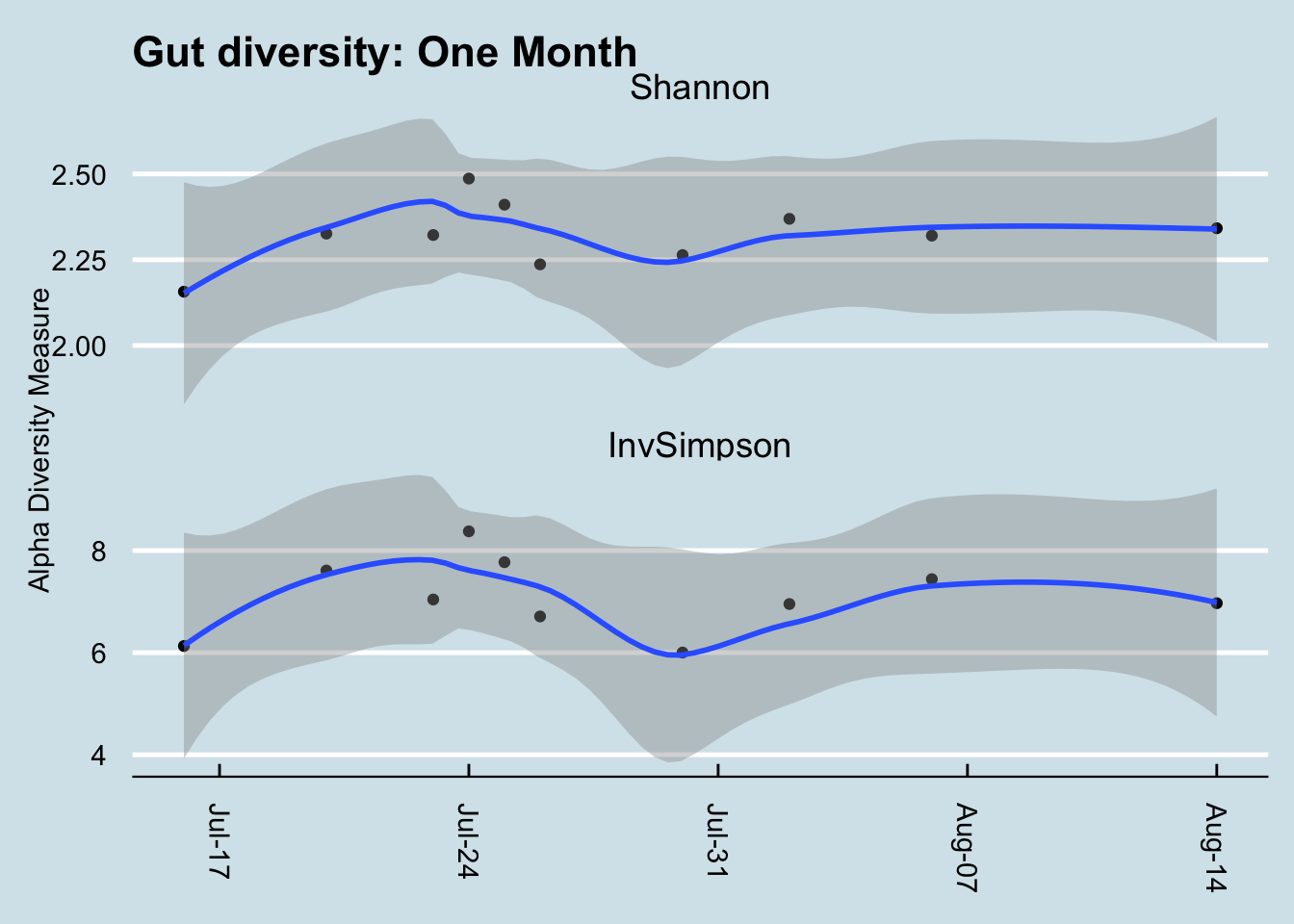
Figure 2.5: Comparing two types of diversity.
That said, Shannon tends to fall within a narrower, more predictable range, so I prefer it over Inverse Simpson when looking at my own samples. It often doesn’t matter which metric you use, though, as long as you’re consistent.
Nevertheless, I have learned to not place much stock in any diversity measure. After all, whether diversity is “good” or “bad” depends on what is in the sample. Is high diversity good even if it includes many known pathogens? Is “low” diversity good if it only includes one or two known commensal bacteria? As always in the microbiome world, it’s hard to tell.
2.4.4 Microbes and behavior*
There are surprising links between microbes and behavior.
Toxoplasma Gondii is a tiny microbe that, for some reason, only likes to reproduce from within the gut of a cat. It can be found in almost all warm-blooded mammals, including humans — about 30% of us, according to some estimates, and that’s after a century of obsession with hygiene that has wiped out countless other tiny inhabitants of the body.
T. Gondii seem harmless because its hosts appear to show no differences before or after “infection” except in one creature: the rat. In this classic experiment71, rodens that were infected with Toxoplasma gondii were studied with special dyes that can show how the infection spreads, first in the gut and then to the brain.
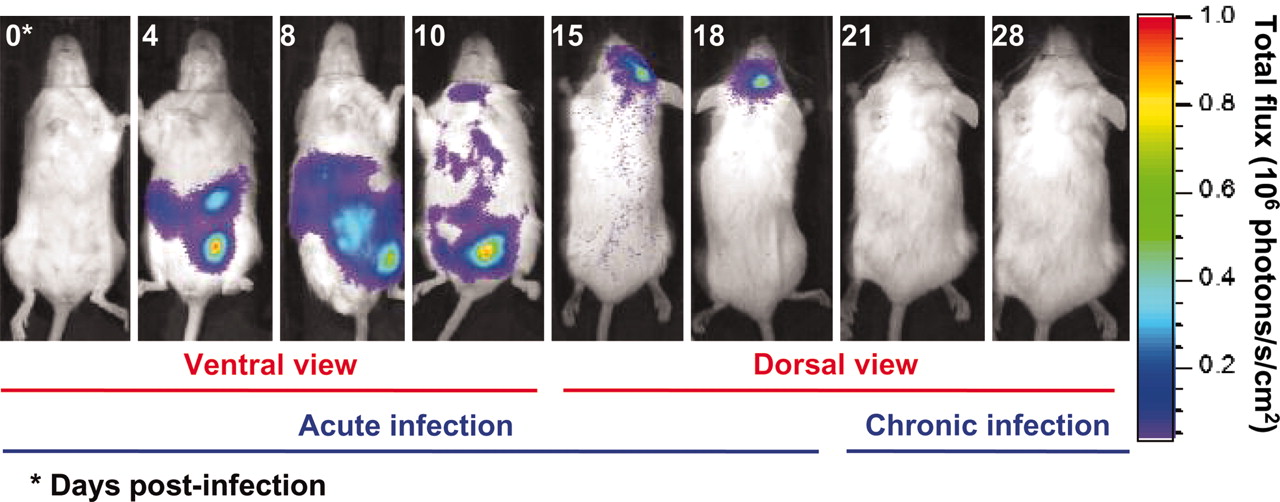
Figure 2.6: From Vyas 2007
When it finds itself ingested by a mouse or rat, it appears to fade away quietly. MRI scans show large amounts in the gut for a week or two, gradually decreasing until there is apparently nothing. By day 18, the last remnants of the infection are disappearing from the brain, except for tiny T. Gondii cysts. Not everywhere, but in just a few strategic places.
Then something strange happens: the rat is now unnaturally attracted to cat urine. And note: its only attraction is to cat urine, not rabbits, not humans — only cats. Everything else about the rat appears normal. It still seems to be afraid of the other things that scare rats: other predators, stressful situations. But put a T. Gondii-affected rodent into a maze with different animal urine in the corners and it will rush to the cat side every time.
How is it that T. Gondii is able to be so precise in its effects? It seems to affect just rodents, and even then it only apparently makes them attracted to cats. It doesn’t apparently cause any other harm to its hosts, so — somehow — it must have found a resting place right at the spot in the mouse brain that affects its interest in cats.
Although there doesn’t seem to be any major negative consequences to humans hosting T. Gondii, some scientists aren’t sure. One man in particular, Jaroslav Flegr, an evolutionary biologist at Charles University in Prague, thinks he has evidence that women who carry it might be more trusting than those who don’t.
Incidentally, some of the drugs used to treat schizophrenia have been shown to reduce levels of T. Gondii.
T.Gondii isn’t the only microbe known to affect the behavior of its host. A more common example is the rabies virus, which upon infection somehow causes a mammal to be more agitated, more likely to strike out — or bite — other humans, thereby spreading itself.
Or syphilis, the disease spread by Treponema pallidum that causes its host to go insane. The microbe is somehow able to infect the mind of the victim.
In general, sexually transmitted diseases are especially likely to have behavioral consequences. A sexual disease that produces symptoms is unlikely to spread, yet it still requires contact with a new victim. Perhaps the ideal vector is a behavioral change, making the host more likely to come in contact with a new host.
A well-done 2019 study (Valles-Colomer et al. (2019)) found that people suffering from depression have significantly lower levels of two groups of bacteria, Dialister and Coprococcus, possibly due to a potential ability of the gut microbiome to synthesize 3,4-dihydroxyphenylacetic acid, a breakdown product of the neurotransmitter dopamine.
There are many other examples of microbes that appear to affect the brain.
5-HTP is an intermediate molecule between tryptophan and serotonin. It is produced by Candida, Streptococcus, Escherichia, and Enterococcus.
Bacillus and Serratia make dopamine
Escherichia, Bacillus, Saccharomyces make noradrenaline
Lactobacillus can produce acetylcholine
GABA can be produced by Lactobacillus and Bifidobacterium.
Your gut contains 100 million neurons72 – about the same as an entire mouse, incidentally –
Bacteroides Fragilis is depleted in autistic patients. it is a gatekeeper for the immune system [ http://www.ncbi.nlm.nih.gov/pubmed/16009137]
Sutterella may have implications for autism, causes tics ?
Bifidobacterium infantis: see Sudo, Chida for gnobiotic mice that it prevents from becoming stressed.
Mark Lyte and his colleagues, a microbiology team from the Minneapolis Medical Research Foundation, studied the effect of infecting mice with Campylobacter, one of the bacteria implicated in the Walkerton epidemic. The dose of bacteria was high enough to be detected in the intestine, but not so high that the mice developed overt illness. You probably won’t be surprised to learn that the campylobacter-infected mice exhibited more anxiety when navigating a maze than the control mice.
Lactobacillus rhamnosus is lower in pups born to pregnant mothers under stress. Intrigingly, this species is linked to levels of GABA, an important neurotransmitter targeted by anti-anxiety drugs like Valium and Xanax.73 What’s more, in mice the action of these microbes seems modulated by the vagus nerve – mice who have their vagus nerve removed seem to be unaffected by GABA.74
Scientists in Japan characterized the microbiome of 25 anorexia nervosa patients and compared them to healthy controls. The AN patients had a lower amount of total bacteria and specifically, lower amounts of Clostridium coccoides group, C. leptum subgroup, Bacteroides fragilis, and Streptococcus.75
Or consider the bacterium Tropheryma whipplei, the infectious cause of Whipple’s disease. Sufferers often have neurological symptoms like memory loss and odd eye and face movements called oculomasticatory myorhythmia, which indicate that somehow the microbe has invaded the nervous system.
Autism (which we’ll discuss later), is often accompanied by a strange craving for propianate-heavy products like bread. You can see videos showing how rats behave when given too much propionate.
We all know people who seem exceptionally fastidious, some who are diagnosed with Obsessive Compulsive Disorder (OCD). The bacterium Streptococcus become relatively more abundant after hand-washing, so they influence the basal ganglia to do more hand-washing? Is that a coincidence?
Think about this too much, and you’ll end up with the obvious question: what other weird microbes are infecting us right now? Can we explain some of our own behaviors this way? Is there a human equivalent of these infections, driving us to do things we “ordinarily” wouldn’t do? And maybe these microbes are so ubiquitous, teeming all over us and in our brains, maybe there’s no way to even know what “ordinary” or “normal” human behavior is.
References
Valles-Colomer, Mireia, Gwen Falony, Youssef Darzi, Ettje F. Tigchelaar, Jun Wang, Raul Y. Tito, Carmen Schiweck, et al. 2019. “The Neuroactive Potential of the Human Gut Microbiota in Quality of Life and Depression.” Nature Microbiology, February. https://doi.org/10.1038/s41564-018-0337-x.
See a detailed discussion in the New York Times: https://www.nytimes.com/2015/06/28/magazine/can-the-bacteria-in-your-gut-explain-your-mood.html↩︎
http://www.microbiomeinstitute.org/blog/2016/1/7/gut-dysbiosis-in-anorexia-nervosa-patients↩︎